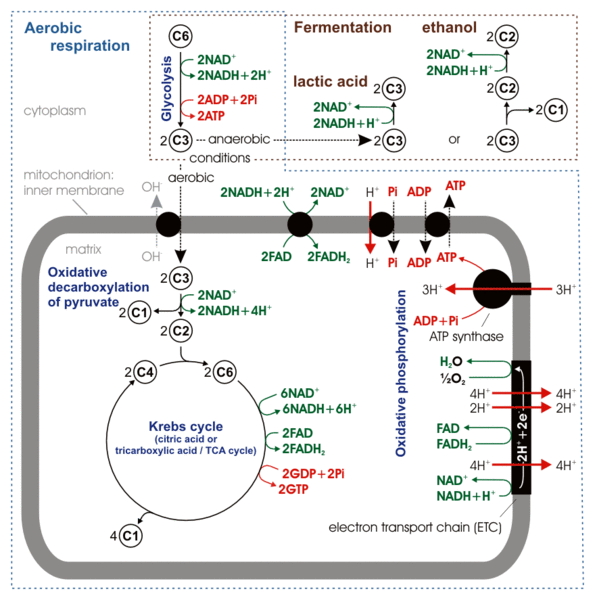
Respiration
Big Picture:
You should review this figure on mitochondria:
Remember that oxidation/reduction (RedOx) reactions involve electrons being traded from positions of high potential energy to ones of lower potential energy, with the resulting release of some free energy (the difference, or delta) between the start and final state.
The overall reaction in respiration combines carbon and hydrogen gotten from carbohydrates and other carbon-containing molecules with oxygen to make CO2 and water. But, instead of doing that all at once, there is a long series of reactions releasing smaller amounts of free energy, some of which is captured in nice, “bite-sized,” chunks of energy we can use. What “bite-sized” chunk really means is electrons in compounds easily oxidized. But, the energy difference has to be small enough to be reversible.
The two main bites of energy for the cell are ATP and NADH.
We will produce ATP through simple reactions called “substrate level phosphorylation” and through oxidative phosphorylation. In this second process, NADH is the source of electrons for the electron transport system, which uses RedOx reactions to pump protons to the inter-membrane space. Finally, the ATP synthase uses that proton gradient to synthesize ATP.
We will start at the end, because it makes more sense this way.
Enter the ATP synthase:
This machine is almost too good to be true. It’s in the mitochondrial inner membrane, the chloroplast inner membrane and the plasma membrane or inner membrane of bacteria. The protons flow down gradient through a channel, which leads to mechanical changes that spin the rotor (more like a ratchet). This mechanical energy is then used to drive ATP synthesis. Thus, we convert mechanical energy to chemical potential energy. The process is also called “chemiosmosis.”
Too cool. Here is a video. It’s not the one from the creationist site.
All this needs to run is a proton gradient. How do you get that? Well, that depends. In the mitochondria and chloroplast, the proton gradient comes from a system called “Electron Transport,” also taking place in the inner membrane of the mitochondria (or chloroplast).
Electron Transport is a series of RedOx reactions taking place in or near the membrane that result in protons being removed from various chemicals and released into a confined space between two membranes (such as between the inner and outer membrane of the mitochondria).
To run electron transport, you need electrons in a high energy state. That is, you need something that has electrons that are relatively easy to remove to a lower state. The thing donating the electrons is the “reducing agent” (it gets oxidized) and therefore we call it a “reducing equivalent” or “reduced cofactor.” It will be one or more “B” vitamin derivatives in its reduced form.
A word about “cofactors.” I hope all of you were starting to see the patterns in the nucleotide cofactors. Take a look at the previous entry on cofactors if you like.
NAD clearly has a lot in common with ADP. NADH has particularly high RedOx potential and is sometimes used directly in enzymatic reactions requiring free energy. These really are variations on a theme. The cofactors in electron transport are variations on another theme, or, pardon the pun, variations on a Heme. These are the iron-containing rings that you are familiar with from Hemoglobin, which can carry oxygen in your blood. But, similar cofactors will be used in electron transport.
If you want to carry electrons in a membrane, you need something that is hydrophobic to do it. The go-to cofactor there is “Coenzyme Q.” It is not based ADP. The “MeO” in the structure stands for a Methyl-Oxygen or “methyl ether.”
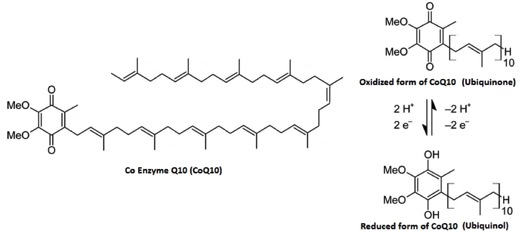
You will see these structures over and over. They will always be doing similar tasks.
Technically, this includes two stages: the phosphorylation by the ATP synthase we’ve already discussed, and the Electron Transport System which uses oxidation/reduction reactions to move protons to the inter-membrane space to drive the ATP synthase.
I stole the figure below from a great text book called “Molecular Cell Biology.”
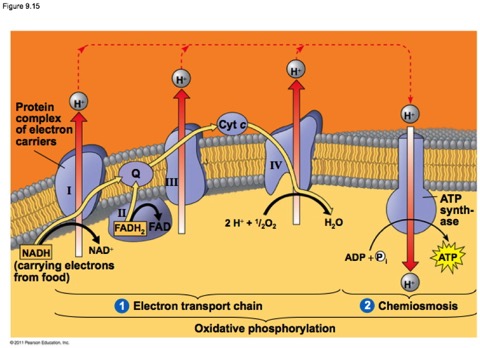
The key players here are called either electron carriers such as Cytochromes and cofactors such as coenzyme Q, or oxido/reductases, enzymes that catalyze the transfer of electrons, frequently while pumping protons across the membrane. You don’t need to know the names of them, though I might use them. The complexes are just labeled with Roman Numerals.
The cytochromes are small-ish proteins that contain bound heme within them. This is cytochrome c, one of the most well characterized cytochromes. Those of you studying cell-death pathways may have run into this one.
The multi-ring structure in the middle is heme and is held in place by the protein. The red dot is an iron.
Technically, the heme is not a cofactor, but a “prosthetic group.” That is fairly subtle distinction. A cofactor generally enters the active site of a protein when it is needed, is altered, then has to be replaced. A prosthetic group stays in the protein continuously. Below are two images of a heme chelating (binding) an iron.
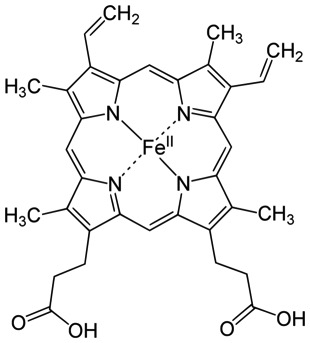
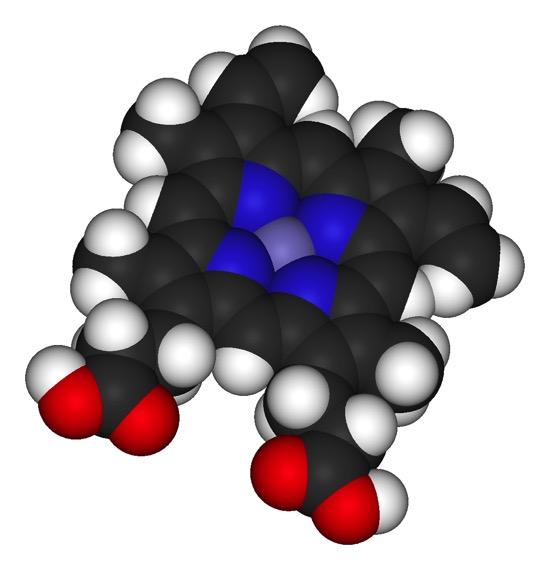
Look at the general scheme on page 171, figure 9.13. Electrons are passed from NADH or FADH2. These enter the “electron transport system” which is the string of cytochromes and other acceptor/donor molecules in the inner membrane. The electrons pass down through a series of RedOx reactions, the acceptor for one step being the donor for the next. Finally, the electrons are passed to Oxygen, which pairs with protons to make water.
At three steps along the way, just due to the orientation in the membrane, the H+ product of the dehydrongenation (oxidation) is spit into the intermembrane space (between the two membranes—we’re so clever with names). This leads to an electrochemical gradient.
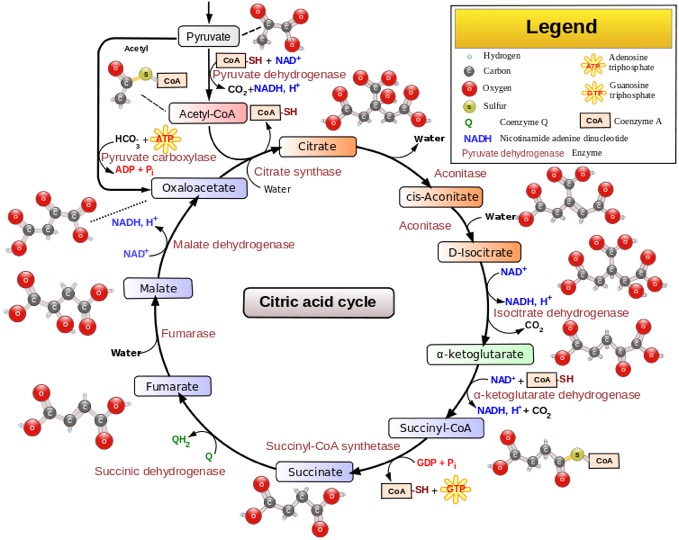
this is also known as the tricarboxilic acid cycle (TCA cycle) or the Krebs cycle, after the person who worked it out.
Yes, I know all the steps and where they connect to the rest of the BSC (Big Scary Chart). No, you don't have to. It occupies the very center of the BSC, for good reason: Even though it is always taught as though it is solely part of respiration, it is really central to all the metabolic pathways. The things made in this cycle are used as building blocks for many other things.
The first thing you need to know about it is that it is a CYCLE as the name says. What that means is that the pathway accepts new carbon units at the top, goes through a mess of steps, and then regenerates the starting molecule, which is ready to accept carbon units again.
The other key things are:
It takes place in the "matrix" or center compartment of the mitochondrion
It oxidizes carbon at several places, which releases CO2 gas…this is the reason you breath out CO2
The electrons taken from the Carbons in this step are passed to the nucleotide cofactors we saw in the Electron Transport System (ETS) today. NADH and FADH2 cary these electrons to the ETS
So—you need a proton gradient to drive the ATP synthase (chemiosmosis). That proton gradient could come from anywhere…but in all the cases we will examine, it comes from ETS…a series of RedOx reactions taking place in or near the inner membrane of the mitochondrion and passing protons to the inter membrane space. To run this, you need high energy electrons. Where do you get them? Well, could be anywhere…but in this case, it's electrons taken from carbon atoms in the Citric Acid cycle and carried to the ETS by NADH and FADH2.
Where does the new carbon come from? Well, in this case, from the food you eat. Could be glucose. But could also be any other carbon- and oxygen-containing food molecules, which is all the things you eat that are actually food.
That's really all the important stuff. Now for some more details.
This is pyruvate,
. It is an end product of breaking down glucose (glycolysis). But, it can also come from other places. It has a carboxyl at the end (that thing at the right). Actually, it is de-protonated in this form, which is why it is pyruvate instead of "pyruvic acid. The carboxyl gets removed as CO2, a “waste product.” That’s an oxidation and the thing that gets reduced is NAD+, yielding NADH + H+ (NADH plus a free proton). As part of the same reaction, the remaining acetyl group (the remaining two carbons with a C=O on the end) is transferred to the cofactor “Coenzyme A” or “CoA” for short. We looked at that a little in the previous post. Acetyl CoA will be an important source of building blocks for other things. Here, it is used to transfer the acetyl to a 4-carbon molecule called oxaloacetate (Remember "OAA" in the BASS 2 activity?) Here it again is shown in its protonated form.
. Adding two carbons to the 4-carbon OAA makes Citrate (or "citric Acid), a tricarboxylic acid:
with a total of 6 carbons. Here it is shown deprotonated with resonant bonds from the carbons to the oxygens)
This is going to go through a series of steps that will regenerate oxaloacetate. A CO2 molecule will come off in both step three and four. Again, this is a net oxidation of the carbon. The oxidizing agent (the thing reduced) is NAD+, yielding NADH plus a free proton. Step five is called "substrate level phosphorylation" producing GTP. All this means is that there is a simple kinase reaction…but it is running in the backwards direction to what we usually think of. Normally, kinases take a phosphate from ATP and transfer it onto some target. In this case, the enzyme takes a phosphate from solution and transfers it to GTP. In the book, it looks like this immediately transfers its phosphate to ADP to make ATP. It can. But GTP is useful itself in many places. Keep that in mind. GTP will be a major player in regulating enzymatic pathways. Two more steps of import: reduction of FAD
(Flavin adenine dinucleotide...the flavin (top left) is not technically a nucleotide...so the name is not quite right. ADP is the bottom right half). It works much like NAD. It gets reduced (gains electrons) and can spit them back out into Oxidative Phosphorylation. Finally, there is 1 last reduction of NAD+ to NADH + H+, regenerating oxaloacetate.
Glycolysis…we'll cover that next.

You may notice that many of these steps require magnesium ions. We should talk some time in general terms about the role of cations in enzymology.
Hexose Kinase (1st step), product is glucose 6-phosphate, keeps glucose inside cell and keeps gradient “downhill” into the cell. This uses an ATP, but is not regulated.
2nd step rearranges it to fructose 6-phosphate. That is, the hydroxyl of position 2 oxidizes the carbonyl on position 1. Position 1 gets the H to make a hydroxyl there and position 2 becomes a carbonyl. The enzyme can be called “hexose-phosphate isomerase” or, as it is on the diagram, phosphoglucose isomerase.
This next step is the rate-limiting and tightly regulated step. Phosphofructokinase (3rd step). This adds a second phosphate to fructose 6-phosphate, making fructose 1,6-bisphosphate. The regulation is via an allosteric site that binds ATP. Now, you have to know two things here: the enzyme has 2 sites that bind ATP, one in the active where it is necessary as a substrate for the reaction and a second that inhibits the reaction; the first site binds with higher affinity than the second. So, if the concentration of ATP is low to average in the cytoplasm, only the active site binds ATP. However, if the concentration of ATP gets high, indicating that more ATP is really not needed, the second, inhibitory site becomes occupied and shuts the enzyme off.
Aldolase (4, clips the six-carbon sugar diphosphate into 2 Triose phosphates called dihydroxyacetone phosphate and glyceraldehyde 3-phosphate (G3P). G3P is the more useful one and the two are freely interconverted by triose isomerase (there are those who call it…"TIM?"). Since there are two, G3P made, all the subsequent steps can be done twice for each glucose).
The next step is carried out by Triose Phosphate Dehydrogenase (takes hydrogen off a triose phosphate). Some charts label this "Glyceraldehyde phosphate dehydrogenase." Glyceraldehyde phosphate is a triose phosphate.
If something is “dehydrogenated” it is the same as being oxidized. The thing that is reduced is NAD+. If you are interested in the chemistry here, it is kind of cool. Phosphate free in solution enzymatically attacks position 1, the carbonyl. The exchange results a carboxyl with a phosphate on it. That’s a really high-energy phosphate. Hydrogen from the carbonyl carbon is transferred to NAD+, reducing it. This is the first energy payoff in that it creates two NADH + H+ (one for each of the G3P), which can be used in oxidative phosphorylation or as a high-energy cofactor in certain other reactions. But, as we'll see later, this reduction can present a problem.
The phosphate on position 1 doesn’t stay long. It is transferred to ADP to make ATP in the next step. This process is called “substrate-level phosphorylation,” and is carried out in this case by the enzyme phosphoglycerate kinase. Those of you learning how the names work might say: “Wait…shouldn’t that name mean it ADDS a phosphate to 3-phosphoglycerate?” How clever of you to notice. The enzyme is named for the reverse reaction to the one we are talking about as part of glycolysis. There are several key enzymes for which this is the case. It turns out that if you purify it away from everything else, it seems to favor the reverse reaction. It can drive it either way. The reason the reaction goes primarily toward producing phosphoglycerate is because there IS a next step. You see, as soon as 3-phosphoglycerate is formed, it gets pulled into that step.
Next is a simple rearrangement where the phosphate gets moved from position 3 to position 2.
2-phosphoglycerate then undergoes a step carried out by a fairly famous enzyme: Enolase. This enzyme pulls a water molecule out but makes the most unstable, highest energy molecule in the series, Phosphoenolpyruvate (PEP). Enolase is essential in every cell that does glycolysis. It is rather famously inhibited by fluoride ions. This is why we put fluoride in toothpaste (and, in less conspiracy-minded communities, in the water). The fluoride kills the bacteria in your mouth. Anyway, that phosphate next to the double bond has to go. It's way too unstable. The enzyme that does that is another really well known one called pyruvate kinase (again, named for the favored reverse reaction). It takes that phosphate and transfers it onto ADP to make one more ATP (well, two more, because we start this portion with two triose phosphates). The product is pyruvate, which goes off to the mitochondria for the TCA cycle.
You should review this figure on mitochondria:
Remember that oxidation/reduction (RedOx) reactions involve electrons being traded from positions of high potential energy to ones of lower potential energy, with the resulting release of some free energy (the difference, or delta) between the start and final state.
The overall reaction in respiration combines carbon and hydrogen gotten from carbohydrates and other carbon-containing molecules with oxygen to make CO2 and water. But, instead of doing that all at once, there is a long series of reactions releasing smaller amounts of free energy, some of which is captured in nice, “bite-sized,” chunks of energy we can use. What “bite-sized” chunk really means is electrons in compounds easily oxidized. But, the energy difference has to be small enough to be reversible.
The two main bites of energy for the cell are ATP and NADH.
We will produce ATP through simple reactions called “substrate level phosphorylation” and through oxidative phosphorylation. In this second process, NADH is the source of electrons for the electron transport system, which uses RedOx reactions to pump protons to the inter-membrane space. Finally, the ATP synthase uses that proton gradient to synthesize ATP.
We will start at the end, because it makes more sense this way.
ATP Synthase
Enter the ATP synthase:
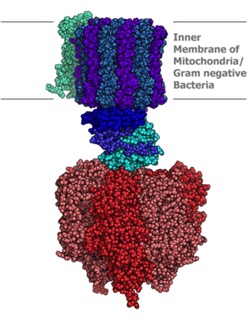
Too cool. Here is a video. It’s not the one from the creationist site.
All this needs to run is a proton gradient. How do you get that? Well, that depends. In the mitochondria and chloroplast, the proton gradient comes from a system called “Electron Transport,” also taking place in the inner membrane of the mitochondria (or chloroplast).
Electron Transport is a series of RedOx reactions taking place in or near the membrane that result in protons being removed from various chemicals and released into a confined space between two membranes (such as between the inner and outer membrane of the mitochondria).
Reducing equivalents or “Reduced Cofactors”
To run electron transport, you need electrons in a high energy state. That is, you need something that has electrons that are relatively easy to remove to a lower state. The thing donating the electrons is the “reducing agent” (it gets oxidized) and therefore we call it a “reducing equivalent” or “reduced cofactor.” It will be one or more “B” vitamin derivatives in its reduced form.
A word about “cofactors.” I hope all of you were starting to see the patterns in the nucleotide cofactors. Take a look at the previous entry on cofactors if you like.
NAD clearly has a lot in common with ADP. NADH has particularly high RedOx potential and is sometimes used directly in enzymatic reactions requiring free energy. These really are variations on a theme. The cofactors in electron transport are variations on another theme, or, pardon the pun, variations on a Heme. These are the iron-containing rings that you are familiar with from Hemoglobin, which can carry oxygen in your blood. But, similar cofactors will be used in electron transport.
If you want to carry electrons in a membrane, you need something that is hydrophobic to do it. The go-to cofactor there is “Coenzyme Q.” It is not based ADP. The “MeO” in the structure stands for a Methyl-Oxygen or “methyl ether.”
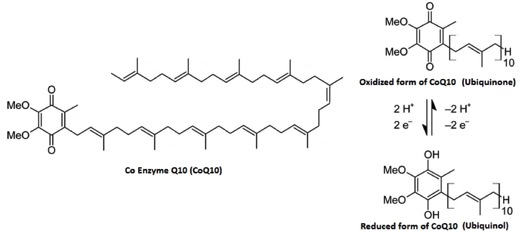
You will see these structures over and over. They will always be doing similar tasks.
Oxidative phosphorylation.
Technically, this includes two stages: the phosphorylation by the ATP synthase we’ve already discussed, and the Electron Transport System which uses oxidation/reduction reactions to move protons to the inter-membrane space to drive the ATP synthase.
I stole the figure below from a great text book called “Molecular Cell Biology.”
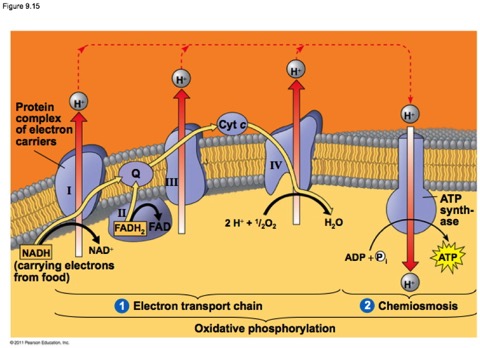
The key players here are called either electron carriers such as Cytochromes and cofactors such as coenzyme Q, or oxido/reductases, enzymes that catalyze the transfer of electrons, frequently while pumping protons across the membrane. You don’t need to know the names of them, though I might use them. The complexes are just labeled with Roman Numerals.
The cytochromes are small-ish proteins that contain bound heme within them. This is cytochrome c, one of the most well characterized cytochromes. Those of you studying cell-death pathways may have run into this one.
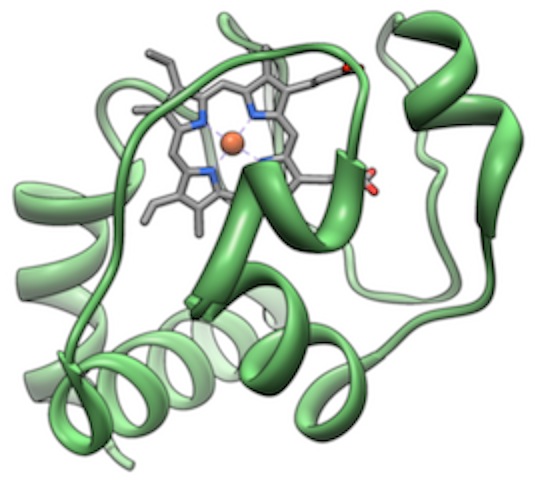
The multi-ring structure in the middle is heme and is held in place by the protein. The red dot is an iron.
Technically, the heme is not a cofactor, but a “prosthetic group.” That is fairly subtle distinction. A cofactor generally enters the active site of a protein when it is needed, is altered, then has to be replaced. A prosthetic group stays in the protein continuously. Below are two images of a heme chelating (binding) an iron.
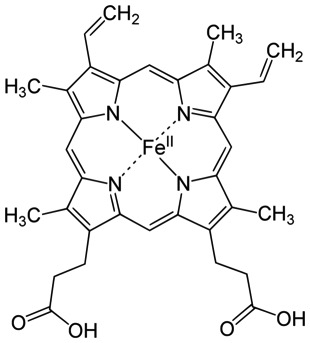
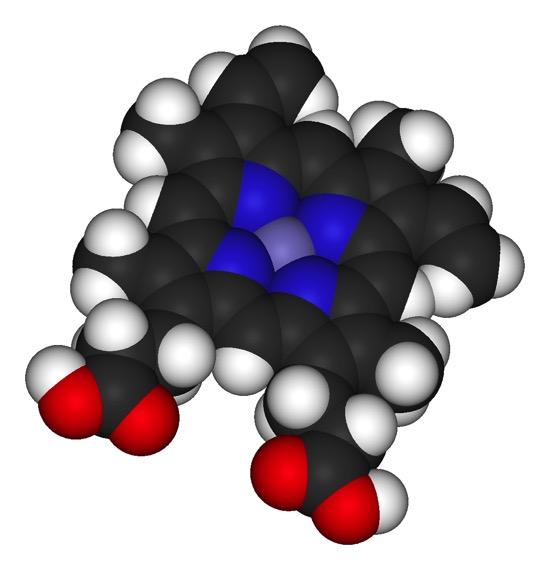
Look at the general scheme on page 171, figure 9.13. Electrons are passed from NADH or FADH2. These enter the “electron transport system” which is the string of cytochromes and other acceptor/donor molecules in the inner membrane. The electrons pass down through a series of RedOx reactions, the acceptor for one step being the donor for the next. Finally, the electrons are passed to Oxygen, which pairs with protons to make water.
At three steps along the way, just due to the orientation in the membrane, the H+ product of the dehydrongenation (oxidation) is spit into the intermembrane space (between the two membranes—we’re so clever with names). This leads to an electrochemical gradient.
Key Points:
- The donor of electrons are cofactors NADH and FADH2 .
- The oxidation/reduction reactions pass electrons from one carrier to another in or near the inner membrane.
- The reactions result in protons being pumped to the inter-membrane space, establishing the electrochemical gradient that runs the ATP Synthase.
- The final recipient of the electrons is oxygen, which combines with H+ to make water. That’s why you breath out water.
Citric Acid cycle
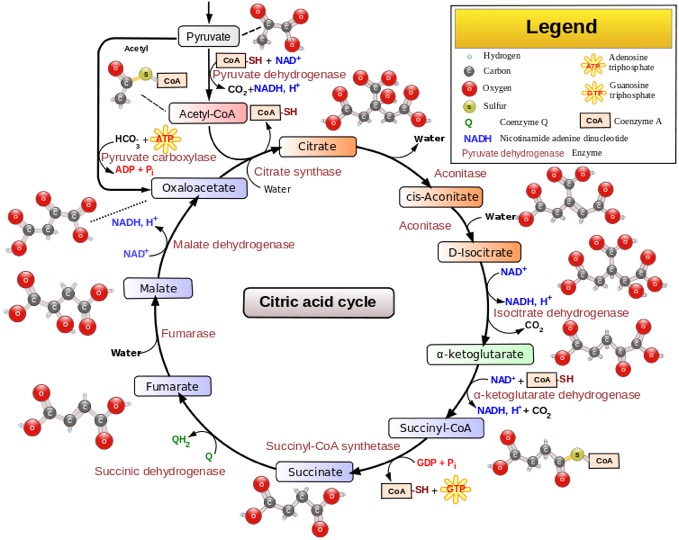
this is also known as the tricarboxilic acid cycle (TCA cycle) or the Krebs cycle, after the person who worked it out.
Yes, I know all the steps and where they connect to the rest of the BSC (Big Scary Chart). No, you don't have to. It occupies the very center of the BSC, for good reason: Even though it is always taught as though it is solely part of respiration, it is really central to all the metabolic pathways. The things made in this cycle are used as building blocks for many other things.
The first thing you need to know about it is that it is a CYCLE as the name says. What that means is that the pathway accepts new carbon units at the top, goes through a mess of steps, and then regenerates the starting molecule, which is ready to accept carbon units again.
The other key things are:
It takes place in the "matrix" or center compartment of the mitochondrion
It oxidizes carbon at several places, which releases CO2 gas…this is the reason you breath out CO2
The electrons taken from the Carbons in this step are passed to the nucleotide cofactors we saw in the Electron Transport System (ETS) today. NADH and FADH2 cary these electrons to the ETS
So—you need a proton gradient to drive the ATP synthase (chemiosmosis). That proton gradient could come from anywhere…but in all the cases we will examine, it comes from ETS…a series of RedOx reactions taking place in or near the inner membrane of the mitochondrion and passing protons to the inter membrane space. To run this, you need high energy electrons. Where do you get them? Well, could be anywhere…but in this case, it's electrons taken from carbon atoms in the Citric Acid cycle and carried to the ETS by NADH and FADH2.
Where does the new carbon come from? Well, in this case, from the food you eat. Could be glucose. But could also be any other carbon- and oxygen-containing food molecules, which is all the things you eat that are actually food.
That's really all the important stuff. Now for some more details.
This is pyruvate,
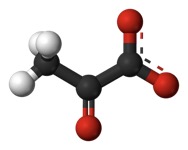
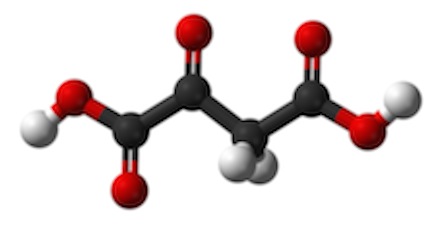
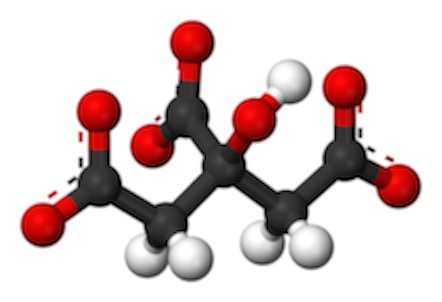
This is going to go through a series of steps that will regenerate oxaloacetate. A CO2 molecule will come off in both step three and four. Again, this is a net oxidation of the carbon. The oxidizing agent (the thing reduced) is NAD+, yielding NADH plus a free proton. Step five is called "substrate level phosphorylation" producing GTP. All this means is that there is a simple kinase reaction…but it is running in the backwards direction to what we usually think of. Normally, kinases take a phosphate from ATP and transfer it onto some target. In this case, the enzyme takes a phosphate from solution and transfers it to GTP. In the book, it looks like this immediately transfers its phosphate to ADP to make ATP. It can. But GTP is useful itself in many places. Keep that in mind. GTP will be a major player in regulating enzymatic pathways. Two more steps of import: reduction of FAD

Glycolysis…we'll cover that next.

You may notice that many of these steps require magnesium ions. We should talk some time in general terms about the role of cations in enzymology.
Glycolysis,
Key steps in glycolysis are:Hexose Kinase (1st step), product is glucose 6-phosphate, keeps glucose inside cell and keeps gradient “downhill” into the cell. This uses an ATP, but is not regulated.
2nd step rearranges it to fructose 6-phosphate. That is, the hydroxyl of position 2 oxidizes the carbonyl on position 1. Position 1 gets the H to make a hydroxyl there and position 2 becomes a carbonyl. The enzyme can be called “hexose-phosphate isomerase” or, as it is on the diagram, phosphoglucose isomerase.
This next step is the rate-limiting and tightly regulated step. Phosphofructokinase (3rd step). This adds a second phosphate to fructose 6-phosphate, making fructose 1,6-bisphosphate. The regulation is via an allosteric site that binds ATP. Now, you have to know two things here: the enzyme has 2 sites that bind ATP, one in the active where it is necessary as a substrate for the reaction and a second that inhibits the reaction; the first site binds with higher affinity than the second. So, if the concentration of ATP is low to average in the cytoplasm, only the active site binds ATP. However, if the concentration of ATP gets high, indicating that more ATP is really not needed, the second, inhibitory site becomes occupied and shuts the enzyme off.
Aldolase (4, clips the six-carbon sugar diphosphate into 2 Triose phosphates called dihydroxyacetone phosphate and glyceraldehyde 3-phosphate (G3P). G3P is the more useful one and the two are freely interconverted by triose isomerase (there are those who call it…"TIM?"). Since there are two, G3P made, all the subsequent steps can be done twice for each glucose).
The next step is carried out by Triose Phosphate Dehydrogenase (takes hydrogen off a triose phosphate). Some charts label this "Glyceraldehyde phosphate dehydrogenase." Glyceraldehyde phosphate is a triose phosphate.
If something is “dehydrogenated” it is the same as being oxidized. The thing that is reduced is NAD+. If you are interested in the chemistry here, it is kind of cool. Phosphate free in solution enzymatically attacks position 1, the carbonyl. The exchange results a carboxyl with a phosphate on it. That’s a really high-energy phosphate. Hydrogen from the carbonyl carbon is transferred to NAD+, reducing it. This is the first energy payoff in that it creates two NADH + H+ (one for each of the G3P), which can be used in oxidative phosphorylation or as a high-energy cofactor in certain other reactions. But, as we'll see later, this reduction can present a problem.
The phosphate on position 1 doesn’t stay long. It is transferred to ADP to make ATP in the next step. This process is called “substrate-level phosphorylation,” and is carried out in this case by the enzyme phosphoglycerate kinase. Those of you learning how the names work might say: “Wait…shouldn’t that name mean it ADDS a phosphate to 3-phosphoglycerate?” How clever of you to notice. The enzyme is named for the reverse reaction to the one we are talking about as part of glycolysis. There are several key enzymes for which this is the case. It turns out that if you purify it away from everything else, it seems to favor the reverse reaction. It can drive it either way. The reason the reaction goes primarily toward producing phosphoglycerate is because there IS a next step. You see, as soon as 3-phosphoglycerate is formed, it gets pulled into that step.
Next is a simple rearrangement where the phosphate gets moved from position 3 to position 2.
2-phosphoglycerate then undergoes a step carried out by a fairly famous enzyme: Enolase. This enzyme pulls a water molecule out but makes the most unstable, highest energy molecule in the series, Phosphoenolpyruvate (PEP). Enolase is essential in every cell that does glycolysis. It is rather famously inhibited by fluoride ions. This is why we put fluoride in toothpaste (and, in less conspiracy-minded communities, in the water). The fluoride kills the bacteria in your mouth. Anyway, that phosphate next to the double bond has to go. It's way too unstable. The enzyme that does that is another really well known one called pyruvate kinase (again, named for the favored reverse reaction). It takes that phosphate and transfers it onto ADP to make one more ATP (well, two more, because we start this portion with two triose phosphates). The product is pyruvate, which goes off to the mitochondria for the TCA cycle.